Stable and high-performing, the new recombinant trypsin product TrypsiNNex® is a reliable reagent for the cleavage of insulin precursors
A brief history of insulin
First isolated from the pancreas of dogs and cows by researchers at the University of Toronto over 100 years ago, insulin is the foundation of diabetes management and, more recently, a component of selected biopharmaceutical processing. At the time of its discovery, insulin was hailed as a medical miracle, and demand for the life-saving enzyme quickly exceeded the production capacity of the Toronto team. A collaboration between influential actors in the pharmaceutical industry and the introduction of isoelectric precipitation subsequently enabled commercial-scale extraction from bovine and porcine pancreatic tissue.1
Early animal-sourced insulin production soon fell short of medical needs. The extraction process was tedious and outstripped the supply of pancreatic tissue. The preparation contained proinsulin, glucagon, somatostatin, and low levels of proteases that could cause adverse reactions in patients.1,2 Even after deploying large chromatographic systems to produce high-purity insulin isolates,2 porcine and bovine insulin remained suboptimal. A single and 3 amino acid difference from native human insulin, respectively, made these animal products weakly immunogenic.
Endogenous insulin synthesis
- Insulin consists of 51 amino acids in 2 peptide chains (A & B) held together by disulfide bonds.
- Beta cells of the pancreas synthesize a single-chain precursor called proinsulin. The long, inert protein then folds, bringing the 2 end peptide chains close enough together to form disulfide bridges.
- Subsequent protease cleavage removes the central portion of proinsulin (the C-peptide) and 2 pairs of end amino acids to produce active insulin.
Trypsin: a game-changer in insulin production
In the late 1970s, trypsin made its debut in insulin production. Novo Nordisk developed a process where trypsin catalyzed a transpeptidation that resolved the single amino acid difference between porcine and human insulin, effectively converting the first into the latter. Under the right conditions, trypsin substituted the terminal alanine residue on the B chain of porcine insulin with the threonine found in human insulin.3 Followed by a chromatographic purification step, the process had a 97% yield of human monocomponent insulin from a porcine source.4
With the advent of recombinant DNA technology, however, a race ensued to create a direct, pure, and scalable human insulin source.1 In 1982, human insulin became the first animal protein to be expressed in bacteria and approved by a regulatory body for general medical use. Today, numerous recombinant insulin variants – from immediate to ultralong-acting enzymes – are used in healthcare and biopharmaceutical production.5
In 1978, Genentech created the first recombinant insulin.7 Two years later, they could produce the molecule at the scale needed to meet demand.8 They used a two-chain method whereby bacteria transformed with the A or B chain sequence expressed the corresponding peptides in separate fermentations, and these were then combined to induce folding and binding.4 Today, however, the dominant production method uses a simpler single-chain strategy. Transformed bacteria or yeast express an engineered insulin precursor where the A and B chains are fused directly or via a synthetic C-peptide.2 The subsequent conversion into active insulin can involve proteolytic excision from the precursor (e.g., Lispro),2 a transpeptidation step, or enzymatic cleavage of the C-peptide.7 Regardless of the processing mechanism, trypsin is the enzyme of choice to catalyze the conversion of recombinant insulin into its active form due to its cost-effective and well-understood performance.
TrypsiNNex® brings consistency into the picture
A paramount aspect of insulin manufacturing is product consistency. The presence of impurities reduces production yields and poses a potential safety risk for end users.9 Variations in process output can cause costly production delays while the causes of deviations are investigated and corrected. Therefore, manufacturing best practices call for using high-quality and homogeneous raw materials to minimize fluctuations introduced by inputs. Trypsin, however, is relatively unstable as it self-degrades via autolysis. In fact, commercial trypsins are mixtures of fully active β-trypsin, subpar α-trypsin, and other components that can differ from lot to lot and change over time. The trypsin used to catalyze the activation of insulin must deliver predictable and consistent proteolytic activity. TrypsiNNex® is a new recombinant trypsin designed precisely to meet these specifications.
TrysiNNex® comprises a homogenous trypsin composition
TrypsiNNex® is expressed in Escherichia coli as an inactive protein. Downstream processing activates the enzyme during the purification step, which is shortly followed by stabilization. Consequently, premature degradation of the active enzyme is minimized, keeping most molecules intact from autolysis. The final product of this manufacturing strategy is more homogenous than other commercial products and contains at least 20% more active β-trypsin, relative to total reversed-phase high-performance liquid chromatography (RP-HPLC) performance at 215 (Figure 1).
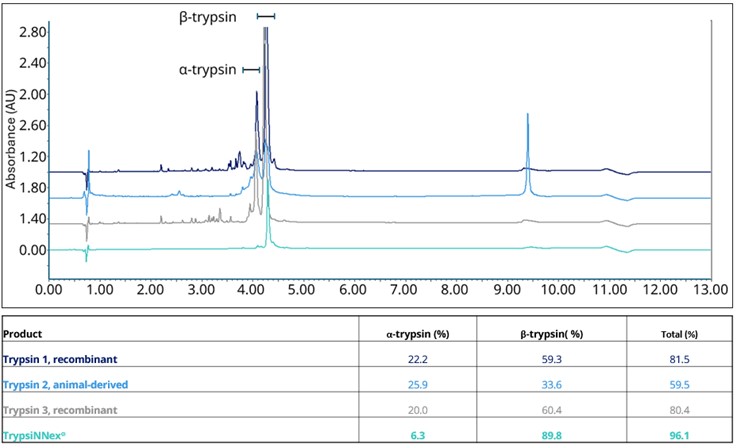
Stable composition and activity
A working solution of TrypsiNNex® with a concentration of 70 U/mL and stored at 4°C retains more intact β-trypsin than α-trypsin for more than 6 months. The initial ratio of β-trypsin to α-trypsin was stable for approximately 30 days before showing the first signs of degradation (Figure 2A). This compositional consistency is reflected in the proteolytic activity of TrypsiNNex®. Overall activity declines only 12% over 6 months (Figure 2B).
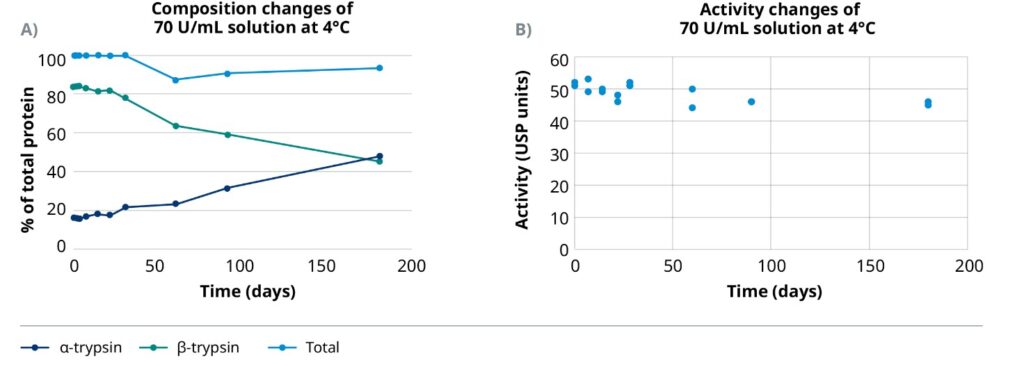
A reliable, fit-for-purpose raw material
TrypsiNNex® is a high-quality recombinant enzyme that addresses critical raw material specifications for biopharmaceutical processing in the recombinant insulin production. Its unique manufacture mitigates premature autolysis, safeguarding the compositional purity of TrypsiNNex® and ensuring top proteolytic performance. This compositional and functional stability enhances process consistency in the conversion of precursor insulin into active molecules via enzymatic cleavage or transpeptidation. Additionally, Novo Nordisk Pharmatech supplies TrypsiNNex® with a comprehensive documentation package for product qualification facilitating a smooth transition away from porcine or bovine trypsin or conversion from another recombinant source, in alignment with industry standards for non-animal origin raw materials. These attributes make TrypsiNNex® a reliable and flexible choice for biopharmaceutical processes including recombinant insulin production, offering purity, stability, and performance in insulin manufacturing.
References
- Sims, E.K. et al. 2021. 100 years of insulin: celebrating the past, present and future of diabetes therapy. Nature Medicine 27: 1154. doi: 10.1038/s41591-021-01418-2
- Walsh, G. 2005. Therapeutic insulins and their large-scale manufacture. Applied Microbiology and Biotechnology 67: 151. doi: 10.1007/s00253-004-1809-x
- Markussen, J. et al. 1985. Single chain des-(B30) insulin. International Journal of Peptide and Protein Research 26: 70. Doi: 10.1111/j.1399-3011.1985.tb03179.x
- Ladisch, M.R. and Kohlmann, K.L. 1992. Recombinant human insulin. Biotechnology Progress 8: 469. doi: 10.1021/bp00018a001
- Landgraf, W. and Sandow, J. 2016. Recombinant human insulins – clinical efficacy and safety in diabetes therapy. European Journal of Endocrinology 12: 12. doi: 10.17925/EE.2016.12.01.12
- Liu, H. et al. 2013. An efficient trypsin digestion strategy for improving desB30 productivity from recombinant human insulin precursor fusion protein. Process Biochemistry 48: 965. doi: 10.1016/j.procbio.2013.04.004
- Goeddel, D.V. et al. Expression in Escherichia coli of chemically synthesized genes for human insulin. Proceedings of the National Academy of Sciences USA 79: 106. doi: 0.1073/pnas.76.1.106
- Fraser, L. 2016. Cloning insulin. https://www.gene.com/stories/cloning-insulin (accessed March 2024)
- Owens, D.R. et al. 2012. The emergence of biosimilar insulin preparations – a cause for concern? Diabetes Technology and Therapeutics 14: 989. doi: 10.1089/dia.2012.0105